The forces between two electric charges in arbitrary motion are complicated by velocity, acceleration, and time-delay effects. The forces need not even lie along the line joining the two charges!
Suppose a source charge q^\prime is at position \vec r^{\,\prime} with velocity \vec v^{\,\prime} and acceleration \vec{a}^{\,\prime}, and a test charge q is at position \vec r with velocity \vec v and acceleration \vec{a}. Let their separation \vec{\mathcal{R}}= \vec r – \vec r^{\,\prime}. Since electromagnetic “news” travels at light speed \color{blue}c\color{black} = 1 (in natural units), define the earlier retarded time s < t implicitly by t – s = \mathcal{R}[s] / \color{blue}c\color{black} = \mathcal{R}_{\color{red}s}.
Liénard-Wiechert electric and magnetic potentials at test charge q due to source charge q^\prime are
\varphi_{\color{red}t} =\frac{q^\prime_e}{4\pi \mathcal{R}_{\color{red}s} }=\frac{q^\prime}{4\pi \mathcal{R}_{\color{red}s} } \left( \frac{1}{1 – \hat{\mathcal{R} }_{\color{red}s} \cdot \vec v^{\,\prime}_{\color{red}s}} \right), \hspace{1cm} \vec A_{\color{red}t}= \varphi^\prime_{\color{red}s} \vec v^{\,\prime}_{\color{red}s},where the source charge q^\prime is “smeared” to an effective charge q^\prime_e by its motion, and the subscripts indicate evaluation times. If the source charge velocity \vec v^{\,\prime}_{\color{red}s} = 0, then the potentials simplify to the electrostatics limit. Differentiate the potentials to find Maxwell’s electric and magnetic fields
\vec{\mathcal{E}} = – \vec \nabla \varphi – \partial_t \vec A \hspace{1cm} \vec{\mathcal{B}} = \vec \nabla \times \vec A,and the Lorentz force law
\vec F = q \left( \vec{\mathcal E} + \vec v \times \vec{\mathcal B}\right)generalizes Coulomb’s law to
\vec F_{\color{red}t} = q \frac{ q^\prime } { 4 \pi \mathcal{R}^2_{\color{red}s}} \left( \frac{ 1 } {1 – \hat{\mathcal{R}}_{\color{red}s} \cdot \vec{v}^{\,\prime}_{\color{red}s} }\right)^3 \bigg( \frac{}{} (1-v^{\prime 2}_{\color{red}s})(\hat{\mathcal{R}}_{\color{red}s}-\vec{v}^{\,\prime}_{\color{red}s}) + \vec{\mathcal{R}}_{\color{red}s} \times \left((\hat{\mathcal{R}}_{\color{red}s}-\vec{v}^{\,\prime}_{\color{red}s}) \times \vec{a}^{\,\prime}_{\color{red}s} \right) \hspace{3.4cm} + \,\vec v_{\color{red}t} \times \left( \hat{\mathcal{R}}_{\color{red}s} \times \left( (1-v^{\prime 2}_{\color{red}s})(\hat{\mathcal{R}}_{\color{red}s}-\vec{v}^{\,\prime}_{\color{red}s}) + \vec{\mathcal{R}}_{\color{red}s} \times \left((\hat{\mathcal{R}}_{\color{red}s}-\vec{v}^{\,\prime}_{\color{red}s}) \times \vec{a}^{\,\prime}_{\color{red}s} \right) \right) \right) \, \bigg).If the test charge velocity \vec v = \vec 0, then the magnetic terms vanish; if the source charge acceleration \vec{a}^{\,\prime} = \vec 0, then the radiation terms vanish; if, in addition, the source charge velocity \vec v\,^\prime = \vec 0, then the generalized Coulomb’s law reduces to the familiar electrostatic limit. Finally, Newton’s second law with Einstein’s momentum
\vec F = \frac{d\vec p}{dt} = \frac{d}{dt} \frac{ m \vec v }{\sqrt{1 – v^2}} = \frac{ m \vec a}{\sqrt{1 – v^2}} + \frac{ m \vec v}{(1 – v^2)^{3/2}} \vec v \cdot \vec agenerates the motion equations using 3-vectors and lab time (instead of 4-vectors and proper time), succinctly summarizing all of electromagnetism.
Using Mathematica, I eliminated present time t from the motion equations with the substitution t = s + \mathcal{R}_{\color{red}s} and numerically integrated them with respect to the retarded time s for various initial conditions, as in the figure below. Positive and negative charge pairs tugged by non-zero net forces spiral into one another as they radiate away energy and momentum, graphically demonstrating the instability of classical atoms.
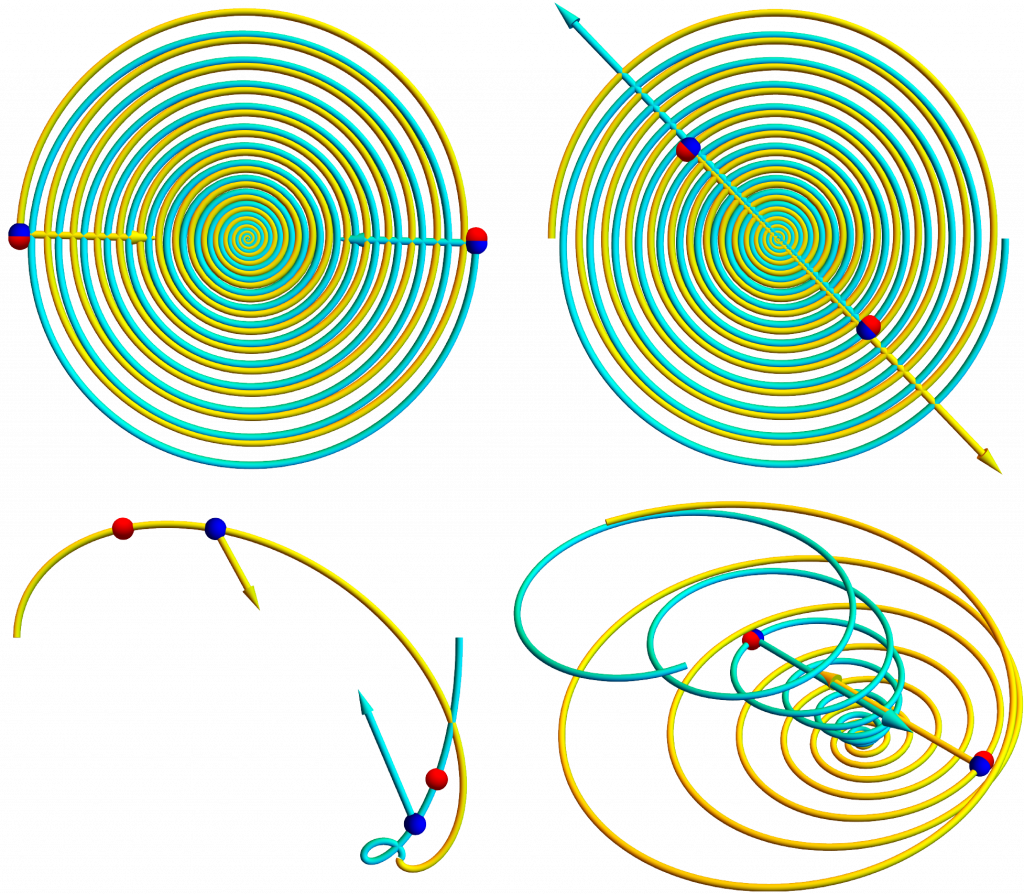
Thanks, Mark! I enjoy reading your posts as well.